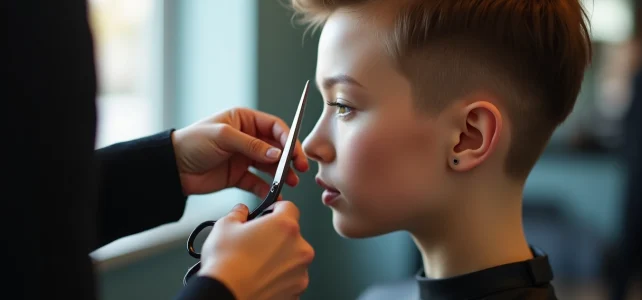
Les salons de coiffure sont en pleine ébullition, révélant des techniques de coupe de cheveux qui repoussent les limites de la créativité. Les experts en coiffure explorent de nouvelles méthodes pour offrir des looks uniques et personnalisés à leurs clients. Des outils de précision aux ciseaux texturisés, chaque détail est minutieusement étudié pour créer des styles à la fois audacieux et élégants.
Les influences viennent de partout : des podiums de la mode aux rues des grandes métropoles, chaque tendance capillaire s’inspire de la diversité culturelle et des innovations technologiques. Les coiffeurs deviennent de véritables artistes, maîtrisant les nuances et les textures pour sublimer chaque chevelure.
Les techniques de coupe et de couleur innovantes
Les coiffeurs sont désormais à la pointe de l’innovation, utilisant des techniques de coupe et de couleur qui redéfinissent les standards de la beauté capillaire. Parmi ces méthodes, le Dégradé progressif se distingue par sa capacité à créer des transitions de longueur harmonieuses et naturelles. Cette technique, réalisée avec des tondeuses modernes, permet d’obtenir des finitions impeccables et des styles variés.
Les coupes et les outils de précision
Certaines coupes, comme les coupes pixie et l’undercut, sont en constante évolution pour offrir une fraîcheur renouvelée à l’image de soi. La coupe verticale est essentielle pour ajouter du volume et de la texture, tandis que le pliage d’oreille permet de travailler les contours de façon subtile, en harmonie avec les traits du visage.
- Balayage : Technique de coloration qui se renouvelle constamment.
- Coupes pixie : Coupes courtes offrant une fraîcheur à l’image de soi.
- Undercut : Technique de coupe offrant une fraîcheur à l’image de soi.
- Coupe verticale : Donne volume et texture aux cheveux.
- Pliage d’oreille : Travailler les contours de façon subtile.
La coloration : un art en perpétuelle réinvention
La tendance automne et automne hiver inclut des techniques de coloration créatives comme le balayage. Ces méthodes permettent de jouer avec les nuances et les reflets pour sublimer chaque chevelure. Les produits capillaires utilisés sont de plus en plus sophistiqués, intégrant des ingrédients naturels pour préserver la santé du cuir chevelu et de la fibre capillaire.
Les coiffeurs, qu’ils soient des artistes reconnus comme Murielle Kabile ou des influenceurs tels que Melanie Nauche, sont à l’avant-garde de ces innovations. Leur créativité et leur maîtrise des nouvelles techniques offrent aux clients des coiffures tendance adaptées à chaque type de chevelure.
Styles et tendances actuelles pour une coupe de cheveux moderne
L’évolution des styles capillaires reflète notre époque, marquée par une recherche constante de nouveauté et de personnalisation. Les coupes de cheveux actuelles se distinguent par leur diversité et leur adaptabilité aux différentes formes de visage. Le bob, par exemple, reste une coupe intemporelle, adoptée par des icônes comme Jennifer Aniston avec sa célèbre coupe ‘Rachel’. La queue de cheval, quant à elle, se réinvente sans cesse, offrant des variations sophistiquées ou décontractées selon les occasions.
Influences de la culture pop et de la mode
Les tendances capillaires sont fortement influencées par des personnalités emblématiques et des événements majeurs. Beyoncé et Jean Paul Gaultier, par exemple, imposent leur vision unique de la coiffure tendance. La Fashion Week, qu’elle soit à Paris ou à New York, continue de dicter les codes en matière de coiffure moderne. Les influenceurs comme Melanie Nauche et Marie Bladt participent aussi à cette dynamique, en partageant leurs créations et inspirations sur les réseaux sociaux.
Techniques et produits en vogue
Les coiffeurs utilisent des techniques avancées pour créer des cheveux tendance. Le dégradé haut, réalisé avec des tondeuses modernes, est prisé pour son look structuré. Les cheveux bouclés bénéficient aussi d’une attention particulière, avec des soins spécifiques pour sublimer chaque boucle. Les produits capillaires à base d’ingrédients naturels sont de plus en plus populaires, préservant la santé du cuir chevelu et de la fibre capillaire.
Personnalisation et adaptabilité
Chaque coupe de cheveux doit être adaptée à la morphologie du visage et à la texture des cheveux. Les coiffeurs, véritables artistes, prennent en compte ces facteurs pour proposer des coiffures sur mesure. Les coupes pixie et l’undercut sont des exemples de coupes qui peuvent être personnalisées pour refléter la personnalité de chacun, tout en suivant les dernières tendances.